131 - Experiments that changed fire science pt. 8 - Modelling Cardington Fire Tests with Asif Usmani
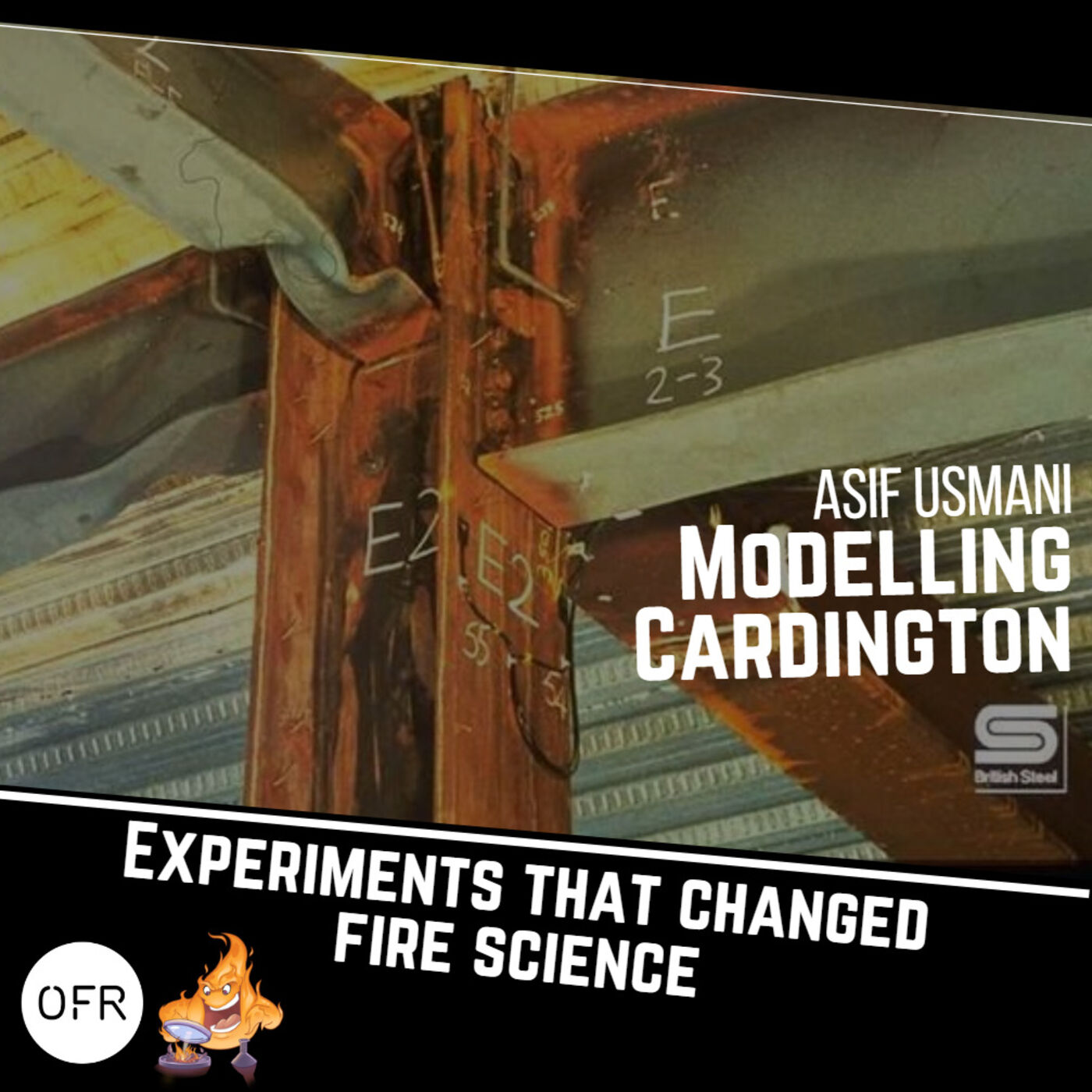
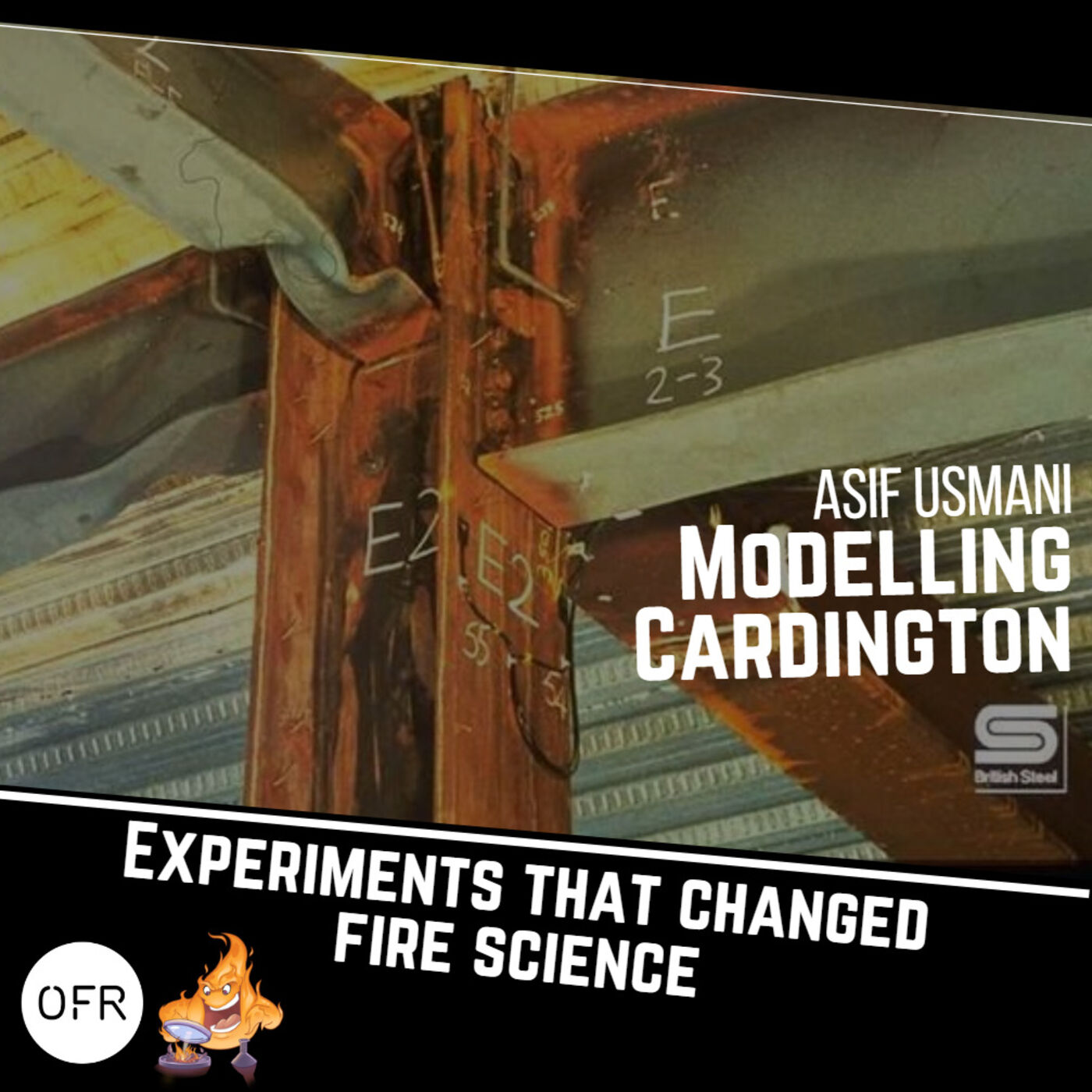
In this part 8 of Experiments that changed fire science series we revisit Cardington (previously covered in part 2 - https://www.firescienceshow.com/078-experiments-that-changed-fire-science-pt-2-bre-cardington-with-tom-lennon/), but this time from the perspective of modeling the structure. My guest prof. Asif Usmani of the HK PolyU takes us on how simplifying the model led them to some fundamental discoveries on the thermo-mechanical response of structures to fires.
We discuss material properties and perhaps their overestimated role in structural modelling. We go into membrane actions and the role of restraints in shaping the response of beams and slabs to thermal loads. And Asif explains to me what this means at a scale of a building frame. Some truly remarkable insights - things that today are perhaps obvious to any structural engineer, but at that time were an unknown fire behaviour.
Here are some links to the papers related to today's episode:
Cardington
https://www.sciencedirect.com/science/article/pii/S0379711201000376
https://www.sciencedirect.com/science/article/pii/S0143974X01000049
https://www.sciencedirect.com/science/article/pii/S0379711204000116
WTC
https://era.ed.ac.uk/handle/1842/1562
OpenSees
https://link.springer.com/article/10.1007/s10694-021-01184-0
https://www.researchgate.net/profile/Xu-Dai-4/publication/283796522_OpenSees-based_integrated_tool_for_modelling_structures_in_fire/links/5647a6b108ae451880ac4f18/OpenSees-based-integrated-tool-for-modelling-structures-in-fire.pdf
Picture credit: British Steel, after "Newman G, Robinson JT and Bailey CG, Fire safe design: A new approach to multi-storey steel-framed buildings, The Steel Construction Institute, Berkshire, 2006"
Accessed through: https://www.researchgate.net/publication/303531122_Shear_panel_component_in_the_vicinity_of_beam-column_connections_in_fire [accessed Dec 06 2023].
Hello and welcome to the Fire Science Show. Today we're back to the series experiments that have changed fire science, and we're back to the very beginning of the series. We have touched the subject of Coddington fire experiments in the early episode of the series, interviewing Dr Tom Lennon from the BRE. But the Coddington was more than just experiments. It also had the modeling counterpart, and today the modeling is what we are gonna discuss. I have invited Professor Asif Usmani, currently at the Hong Kong Polytechnic University, but at the time he was a young postdoc at the University of Edinburgh working under Dougal Drysdale, putting a project under the name of Dougal. This is a really funny and interesting story and such a big chunk of history fire science. But most importantly, they've tried, they've proposed that they will do modeling of the British steel tests of the steel frame building that were just happening at the Coddington at the time. They've rushed to model the entire structure and boy. They've learned a lot of difficult lessons on how the geometrical non-linearities create significant complexities in understanding fire behavior of a structure and they found that a lot of damage done to the structure is actually caused by thermal actions, not just the material properties you know, steel losing its strength due to high temperature, but the completely different set of thermal mechanical actions that act on the structure when it's subject to fire. Such an interesting world to discover. And they were the pioneers the pioneers of modeling structures in fires. So let's jump into the episode and learn how they started it, what they've learned while doing that and how the landscape looks today. Let's go. Welcome to the Firesize show. My name is Vojci Wigzyński and I will be your host. This podcast is brought to you in collaboration with OFR Consultants, a multi-year, award-winning independent consultancy dedicated to addressing fire safety challenges. Ofr is UK's leading fires consultancy. Its globally established team has developed a reputation for preeminent fire engineering expertise, with colleagues working across the world to help protect people, property and environment. Internationally, its work ranges from the Antarctic to the Atacama Desert in Chile, to a number of projects across Africa. Ofr is calling all graduates, as it is opening the graduate application scheme for another year, inviting prospective colleagues to join their team from September 2024. By taking this opportunity, you'll be provided with fantastic practical immersion in the fire engineering and unique opportunity to work with the leading technical experts in the field, while learning the skills critical to become a trusted consultant to clients. This opportunity is tailored just for you and if you would like to take it, please visit OFRConsultantscom for further details and instructions on how to apply. Hello everybody, I'm here today with Professor Asif Usmani from Hong Kong Technical University. Hello Asif, good to have you, Hi.
Speaker 2:nice to see you again after Japan.
Speaker 1:Yeah, Japan was just amazing and I really appreciate to be able to connect to so many people in person. This was the real highlight after all those years of COVID. Anyway, Asif, I know you have been involved in the modeling site of the Cardings project, which we have already covered to some extent on the podcast. Of course, you cannot give justice to Cardington with one hour interview with Tom. I've invited you here because I know on your side you had the modeling and, to my best understanding, Cardington was a huge experimental program, but it was also a giant leap in the way how we model fire response of structures. So I want it all. I want to know what happened and how it happened. So perhaps let's start with how did you get involved in modeling huge experiments?
Speaker 2:Thank you. Thank you for watching. It's a very, very interesting story for me anyway, I had moved from Swansea after my PhD in postdoc etc. Working in finite element methods in Swansea, which is the famous place for finite element If those people who work in finite elements will probably know very well about Swansea and the professor Oseezin Kavich, who was considered the godfather of finite elements. So that was a really nice time of learning about medical modeling and understanding finite elements deeply. And then, as my first lectureship job, first university job, I got an offer at Edinburgh University. So that's when I started, in 1995, january, my lectureship in Edinburgh and as a new young academic I was looking for things to do right To develop my research career, because Edinburgh was not really a finite element place, it was much more of a. You know they were engineering, there were a number of different areas that people were working on, but fire had a very strong background there, very strong legacy Professor David Raspash, who had started it in Edinburgh, and then Professor Dugard Reisdale was there at the time when I joined. So it was quite nice to get together with him. And within a year or so I don't remember the exact date I found out through a newsletter that these carton tests were happening in Cardington and BRE and British Steel were involved. It was a British Steel newsletter or something like that. That's how I kind of found out, because I had no idea there was something like this going on at this big scale in scale. So at the time I think not all the tests had happened. There was, they were going to happen. One or two were going to happen the last ones and so we got in touch with British Steel and asked them. They were interested in the modeling of these tests, because obviously you can learn a lot from tests, but to learn even more and their best value out of these experiments, you really need to then try and see if you can simulate what happened in these experiments and get to the sort of deeper detail of the mechanisms and the mechanics of what's the behavior of the structure under these kind of realistic fires that they were testing. So this is how it started and it's a long story in terms of how we got the project. It took almost a year before we got the project Negotiations, this, that, and there were some issues there. So ultimately we did finally get the money, which was quite a big achievement in terms of for me to be able to get some funding. Actually we applied in name. I remember I wrote the proposal with my colleague Amadal Guzuli, who went to Imperial later on. He was at Edinburgh then but we named Professor Dougal Drysdale as the PI. He's a chemist and he's a fire dynamics expert and his books obviously very famous fire science, fire dynamics. But he is known in fire so and we were unknowns, we had no fire background, we had done nothing before. So just to sort of kind of cover, that kind of weakness played the game of using Dougal's Drysdale name as the PI. So it kind of worked and you know we managed to get it and Dougal Drysdale was involved in the fire, sort of giving us advice on how to look at the fire issues there. But mainly it was a structural project. It was not much fire in it, just the temperature, because the temperatures are measured as well. So we had all the temperature data so we could start, you know, working on trying to see if we could reproduce these tests using finite elements.
Speaker 1:If I had the opportunity, I would put Dougal in my project as well. That's a good choice. Was the initial intention to model the entire building or parts?
Speaker 2:Yes, that's right, very naively. Very naively because we had at least I personally had a lot of finite element experience, a lot of modeling experience, and structures was also quite a strong sort of background in mind. So I kind of naively thought, oh yeah, I'll just make up the whole building and, you know, I'll just apply the temperature history and you know, hey presto, we get all the results very quickly. And then reality came to bite us very badly and we found that almost for a year we had nothing, nothing, nothing. Because this problem is fundamentally so fundamentally nonlinear. And it's not nonlinearity necessarily because of the thermal effects. Just thermal effects produce nonlinearity. Right, if you obviously heat steel and concrete, they both lose their strength and stiffness and you have to take care of that in your material models. You have temperature dependent material models and those are those sort of things we were fine with. But key thing that was killing everything was geometric nonlinearity. It was the deformations that were created because of the, because we didn't want to play any games with, we wanted to, you know, be as true to everything as possible. With the thermal expansion coefficients of the steel and concrete we're adding to the models and as soon as we did that we wouldn't get one converged iteration of the adding this thermal loading. So basically then big models started becoming smaller and smaller and smaller and smaller. They'll be ended up with a beam. We say, okay, just let's do it, let's do a beam and see what happens to that and some restraints or something to just get the effect of some. And I did a lot of analytical work as well, which has been also published in these fundamental principles paper, which is one of the most, which is my most highly cited paper. I think it's more than four, five hundred citations there. So that basically was almost like this first year of total failure in trying to get any meaningful results from the modeling taught us that we have to really take the thermal mechanics aspects, the deformation aspects, these very, very seriously. And we need to understand the theory. We need to understand how a single beam behaves. Simply support a restrained beam, restrained with thermal, you know, with rotational restraints, with translational restraints, all of those things. We try to develop them, basic models, and then we try to go into abacus with single beams and try to get results of those. And and we started then we started getting results and the first British Steel test is actually a restrained beam test which you might, might remember, and that restrained beam test essentially is just a beam, but it's just totally restrained. It's all sides. Obviously, there's a slab connect and there's there's three meter widths of the slab that was heated and eight meter lengths of it up a nine meter beam. So the ends were totally restrained, the structure around which totally just restraining the beam rigidly. Well, the whole whole behavior is totally dominated by this restraint.
Speaker 1:So we need to step back a little bit, because most of my audience are not structural engineers and what you're really saying is very fascinating, but let's give people an idea about what does it mean?
Speaker 2:OK, so let me start. Let me try and explain it simply first. So if you just look at a simple beam like when structural engineers, you know, start learning about structures in university and then they're introduced to a beam, they're typically taught a simply supported beam, so be bending and shear, and these kind of things come into it. Where basically the beam has one side is basically that the pin, pin supports they call it right, so knife edge supports or pin supports or roller supports are called. So one end is on a roller and the other end is sort of restrained from horizontal movement but rotation is free. So there are two degrees of freedom the translation degrees of freedom where the beam can go sideways, the beam edges or it can rotate. So pin support restrains can restrain the translations but doesn't restrain the rotations. But you can have fixed end supports which can restrain both rotations and translations. Ok, so students are initially taught that, but when you are in a real building, real building frame like Cartington situation, you almost have a completely fixed support. You know there's fully restrained. That means you don't have translation at the ends or at least the translation is not infinite support Like in theory. You can have infinite supports where you have just a, just a restraint, which means that if you apply any force to it it can take in any number amount of force without any movement. Ok, that's, that's rigid and that's theoretically impossible. So obviously the reality is that it move a little bit with the push and that would be like a spring support, but very high stiffness of the of the restraint, so it will not allow the beam to move sideways. And the more important restraint is actually the rotational restraint, so the beam. Although we often model the beams as semi-rigid or sometimes something like that steel construction, what happens is that when you have a composite slab which is continuous, continuous composite slab over the connecting column and beams and stuff, and you have a beam connected to it and so the beam connects to at a lower point to the column and then the slab is connected, obviously continuous long, and they are both connected. So this becomes like a very rigid system of two points and that means the rotation is not even free. It's not a knife edge, it's not a pin anymore. So this makes this exceptional sort of very strong restraint. It's not 100% rigid but it's still very solid restraint. And this restraint makes you see so, and as soon as you heat it the steel wants to expand and the difference of temperature creates a gradient. So the temperature in the bottom member of the steel part is much higher than the composite because, if you remember, it was uninsulated. The restraint beam test, the only the ends were insulated. The center was uninsulated. So there's a significant thermal expansion going on in the steel because the temperatures are high. Even at very low temperatures just 150 or so, 150, 200 degrees the bottom flange temperature you get such high forces developing that the bottom flange buckles Yields actually and people call it buckling, local buckling. You must have heard local buckling a lot. I find that inaccurate in a way because it's not local buckling. If you just do a simple calculation this is in my papers you do a very simple calculation and around 150 degrees or something, the force in the bottom flange becomes so high because of this restraint effect that the steel yields Yielding at 150 degrees. So, as I said, low temperature effects are very strong.
Speaker 1:At the point when you approach Cardington. I wanted to ask you what was lacking, but from what you previously described, I've heard that everything was lacking. So this type of behavior was recognized, and just not it was ignored.
Speaker 2:At this time you will not find it any other papers other than our early papers from Cardington, before we started publishing, maybe about a couple of years after we started the project, because the first year, as I said, was a complete learning process and we didn't get any decent results. But after the first year we managed to, when we understood the problem a bit better, then we started to know how to deal with the numerical instabilities that were being caused because of these similar forces that were being generated and we started to understand how to deal with them and we reduced the step times etc. Sometimes we'd use devices like ramping up the thermal expansion coefficient over a small period of temperature in a small time. So initially you start with without thermal coefficient and then you just ramp it up so it gradually builds up instead of hitting it immediately and those kind of tricks that we use. And obviously you need a strong geometrical, non-linear model and that was available in Epochus. That was quite good, but it just was. We found that the non-linearity was too strong. We just had to control that. Once you get through the initial hiccup, then it would follow it much better. I think that starting process was very, very tricky. That's why we didn't get initial results, because we just found that as soon as we started the analysis it used to fail. So that's why we spend a lot of time just trying to see how we can get it to go.
Speaker 1:So trying to understand the modeling components. So the software package that you've used, Apochus, it could do the frame, it could do the, let's say, some thermal mechanical.
Speaker 2:It could do everything. It could do everything.
Speaker 1:It was just the complexity of the set.
Speaker 2:It's just the complexity of the non-linearity that was created by the thermal expansion forces as soon as because you do several load steps you start with that gravity load step and you get converged solution after the gravity load step. But that's mostly linear anyway, so that doesn't cause much problem. And once you get that, then you start applying increments of temperature, because all these temperatures have to be applied in small increments. So that's what the non-linear process is that you don't introduce the whole load at one time because the numerical method will not deal with it. You need to follow, because the load and displacement are not linear. So load and displacement have some sort of non-linear paths and so you have to apply small increments to follow that path. So in this case load is just temperature. So we apply small increments of load and those small increments had to be really small in the beginning to get a converged solution, otherwise it won't converge. So I think this is how we, because mainly because as soon as you apply the temperature increment you'll get internal forces generated because of the thermal expansion and the restraint effect and they would cause a lot of destabilization and you wouldn't get equilibrium. That's how you kind of move into the simulation.
Speaker 1:If we can go back to the structure itself, I'm jumping a little bit with it. It's really interesting and as someone with not such strong structural or finite element modelling background, I need to comprehend this. So, going from beam to frame, those effects you just described in the whole frame they must exist at the level of individual beams, of course, but you have many load bearing paths in your frame. That's right. To what extent these low temperature effects are dangerous for a frame of a whole steel building?
Speaker 2:Yeah, so that is a very pertinent and interesting question. So you may sort of claim to be not so knowledgeable, but you asked a very, very pertinent question. You're being humble. So I think the thing that we learned a lot after, when we had done quite a bit of the Cardington modelling and we'd modelled the whole frames or part frames at least, we did model the whole building ever, we modelled a few bays etc. That sounds like a great project for today. I see, yeah, it was a very good project. So the corner test is like, you see, the string beam test. The first one was just a beam and a small section. The second one was called the frame test, which we didn't model. We thought that was a very unrealistic situation because they had a strip along the frame. That would never be a realistic situation. But the corner test was the third. Which we found most interesting was the third British steel test, which also we learned a lot from. That's where the membrane behaviour came from, so all the membrane effects and stuff we understood from there. So that's an interesting story in itself. So that one we modelled sort of like a neighbouring bay of the corner bay and the top and bottom too, so slightly the effect of the rest of the building. But basically these things are very sort of localised. The rest of the building is not really doing that much, it's mostly just the local area. So if you just model the surrounding structure, I think usually that kind of gives you a recent result. So we could model all those things quite well after those initial experiences. But your question about what it means for big buildings, that's what we started to learn and the low temperature effects is the key point that you made, because I think you picked up on that my point on that. These low temperature effects are very, very important.
Speaker 1:If you're telling me something happens at 100-150 degrees at the bottom, that's right, 100-150 degrees.
Speaker 2:Well, the thing is that it does happen in the restraint beam. It does happen, but it has really almost no consequence to the structure. What it does is just changes the restraint beam into a beam that is still restrained in translation, but not so restrained in rotation. Because, now the steel beam starts to yield so it can rotate, so it can as the bottom starts, that is, to yield. So it's like almost change in the boundary condition. Okay, your boundary condition goes from being fully fixed and rotationally and translationally to being fixed only translationally and rotationally. It becomes free, Okay. And so you get much larger deflection suddenly. So you find that you have slight plateau in the beginning and the temperature deflection results and then plateau just suddenly breaks and then you have a jump in the deflection. The deflection starts increasing much faster Because now your thermal expansion is being accommodated in deflections. And that is where I just make this point, if you mind, is that I mentioned that the material effects are overemphasized. So when people see these high displacements, high deflections in buildings after fire, they automatically assume that these are because the fire has weakened the structure and the structure has lost its strength and stiffness and it's now sagging because of its weakness. But what we found? That there's a majority of it, the vast majority of this is happening in the early stages, where the structure is not weak. It's got most of its strength and stiffness. It's the thermal deformations, Is the thermal definitions that are driving it down, that are driving those deflections through the thermal bowing effect. So at least in both the British Steel's test restraint beam test first test and the third corner test, both situations we found that the thermal expansion was totally dominating. Right to the end of the test the whole slab was in compression in the corner test, except the other side. There's one side is longer, 10 meter by 8 meter is the fire compartment. So 10 meter side is obviously longer, so you get slightly longer deflection. And the other side, the 8 meter side, cannot have the same amount of deflection because it's shorter. So the longer side is pushing it down so that's causing a stretch, so you get some tension in the shorter side. So we show that in our papers. On the corner test is that you see that this kind of interplay of the thermal expansion just driving the whole thing, and it's nowhere near any kind of failure, it's just all effects, even though the temperature in the third test was quite high, almost 1200 the compartment temperature, but still the structure had no real problem dealing with it and the deflections that you see are very much driven by this thermal deformation. And so this is what we learned. Now, how do you then connect it to big general frames? Is when we started to learn from the World Trade Center collapse. So when the World Trade Center collapses happened, then we kind of quite surprised that we saw such good results in Cardington, such good behavior in Cardington. The steel beams were so good. What happened to these buildings? Okay, they had very, very big fires. For sure they didn't have like one small, one small fire.
Speaker 1:There are multiple floors in Saruna.
Speaker 2:Three, four floors are involved in fire multiple floors so it was much bigger fires, obviously, and there's also damage and all that. So we thought, okay, it's obviously much worse situation, but collapsing completely we thought was too much. So we started to investigate this and we got the basic data from the FEMA report that came out very soon after collapse is about a year or so after. So we started working on that and over time we discovered collapse mechanisms which are low temperature collapse mechanisms so you can have especially multiple floor fires. If you have multiple floor fires, this thermal buoying that I'm just talking about, that you see in Cardington, everywhere. What's happening is, you know, in the World Trade Center you have the truss and you have the slab on top and you still have this thermal gradient because the truss is much hotter than the slab and this thermal gradient then causes massive buoying. This is a very long floor span. This is 18 meter floor span. So 18 meter floor span starts thermal bowing, and two or three floors, if they do it together, they start to pull columns in, because if it's going to bow, then it loses length, it gets shorter, so it starts to pull the columns. So either connections break or the column gets deformed and if three or four floors are simultaneously pulling columns in, then you can actually, in some pictures you do see columns bending in actually just before collapse. And this is what we predicted that these and these don't have to be very high temperature. This can happen within five, four, five hundred degrees or something. You could get the frame to completely, the column to collapse and the column temperature in the column. We didn't even heat in this in our models. We said OK, because the World Trade Center columns had three floors outside the building. So if you look at the pictures of the World Trade Center, the three faces of the exterior perimeter columns, yeah, they were the exterior outside. They're outside. Only the one face was inside. And so we said, ok, we won't bother to even heat the columns or heat them at a normal temperature. Just heat the floors, and the floor action just kills the columns.
Speaker 1:You brought me to another interesting subject that I wanted to ask you in this interview Heat transfer. So to what extent the heat transfer in the structure was already solved in the modeling, to what extent it was a challenge on such a complicated level?
Speaker 2:Heat transfer part itself is more complicated, more because of the fire. Yeah, the heat transfer itself is, I guess. Obviously, if you have insulation and stuff then it's a little bit more uncertain as to insulation, conductivity properties and all those kind of things. So those things are a bit more challenging. But most of our analysis that we did, we usually assumed insulation is falling off so we're just heating the steel directly.
Speaker 1:But you didn't have adiabatic surface temperature back then or other tricks to make it easier.
Speaker 2:No, in the Cardington we had temperatures directly on the beam because they had thermocouples. So they had quite, not very fine resolution but sufficient number of points that could give you an idea that if you assume that the fire is relatively uniform in the compartment then you could assume that the whole beam is roughly the same. So you had two or three points over the depth and stuff. So you could get and the slab temperatures were available and the column temperatures were slab temperatures. I think we didn't have on the maybe in the corner test. We probably didn't have that. We probably had a little bit of strength. We can't remember exactly. I think we did have to do some heat transfer analysis also in this Cardington project to try and get detailed temperatures in the slabs, because the slab temperatures were sometimes just only one or two, but the exposure, the fire exposure, was completely uniform over the whole beam or the whole slab, the whole corner. We assumed well at the time we didn't look at the fire exposures specifically, but we just took the temperature data that the Cardington test provided. So there were sufficient temperature data, provided that we could carry on the and we assumed that they were reasonably reliable. So we did it on the basis of that. Where we felt that there were some more temperature information needed, we did heat transfer analysis to try and also try to validate it with some of the known temperatures to get more resolution in our models. So that's, the heat transfer wasn't such a major issue in that project but in the World Trade Center projects. In the World Trade Center we didn't have any money for that, we just did it through PhD students for the World Trade Center. The World Trade Center we just did it out of interest. So there were a number of PhD students. The first paper that's become the most cited one is with a master student who was self-funded. He did quite a good analysis of the World Trade Center and there we did not use any kind of. Obviously nobody knows what the temperatures were, so we just used a whole range of uniform temperatures, like from low temperatures, 500, 800, 900, et cetera. So we had a whole range of and with different power growth rates and different temperature growth rates, et cetera. So we tried to do it through using. If you look at the paper, you will find that we did more than a few hundred analysis of the same frame with different temperatures so we could get you know, hopefully cover the real situation.
Speaker 1:I think it's important because we're in the structural paradigm, we are very reliant on fire resistance and you would also to some extent connect fire resistance with perhaps the worst possible fire. You know, fully developed fire, flash over and everything. However, I've also learned that in many cases a localized fire, some sort of non-uniform fire exposure of the structure, can lead to actually worse outcomes. When we worked on the X1, the first big traveling fire experiment with Imperial Agla was closing her PhD at that point, if I'm not wrong, and she was showing that there was a particular range of traveling fires, which were actually the 10, 20% of the compartment involved, which led to the usually the worst outcomes. So I now kind of intuitively even understand that you never know which fire will be the worst for your structure and its experiments like Cardington that give us that's why.
Speaker 2:That's why we, when we did this World Trade Center work we obviously didn't have natural fires at the time, but we just use sort of like uniform but a whole range of temperatures for the trusses to try and say that, okay, these are. We also tried to do non-uniform, so we did where we said okay, if these are large compartments with 18 meter span, then maybe most of the heating is near the windows. So we kept the temperatures of the windows near the windows very high and then we ramped the temperature down towards the interior. So we tried to do these kind of things. You know, this non-uniform behavior we kind of have tried to also bring in, but not in a sophisticated way in the beginning, but obviously later on we have also followed on to this idea of traveling fires and stuff. We are using that all the time now. I think that's very, very reasonable to have a significant number of realistic fire scenarios for any kind of design, to make sure you're at least picking up, at least have a chance of picking up the worst cases.
Speaker 1:Picking up on also to one of my understanding. One of the very fundamental outcomes of the Coddington was the building. The frame could take much more than it was expected to. The membrane actions that formed that gave much more, let's say, fire resistance to the structure. I'm using fire resistance so incredibly now, but I enjoy it. So I wonder if in your scale of modeling have you also observed similar effects and how it translates again when we go to the full building.
Speaker 2:Yes, that's right. So that's what I mentioned to you about the corner test. That's where we first noticed the membrane action quite strongly. The slab was on the 10-meter span side where the primary beams were. There was deflecting more and it was causing a stretch on the perpendicular side, the 8-meter side. But if we say had the fire gone on for longer? Because what we found from the third test is that this membrane action is still being driven. You see, this is where one of the things that we differ with some of the literature so like, if you look at Colin Bailey's work and some of the other work, they have assumed that these slabs are kind of rotationally unrestrained, so that you can imagine two ways. So you can have a building like a rectangular shape with a slab on top with nothing connecting to it. If it's nothing connecting to it and the slab is just sitting on the beams, then if you have a fire underneath it, the slab still displays membrane action because of the compression ring that you see, because what it has to do is because it has to geometrically deform to get through. You see, because the slab is bigger than the hole it is covering. If you think it like this, it's the hole that it is covering. It is slightly bigger than that. If you take a piece of paper or whatever and you try and fold it through a hole that it's covering, then it has to squash and then go down. So that's squashing effect. So as it deflects more and it pulls in from the sides, then a kind of compression ring forms around the slab and that gives you membrane action. So I think if you look at Colin Bailey's work, he has exploited that effect and he applied it to Cardington and we have published papers also in that area where we have sort of said that that kind of model is fine in a kind of design sense. So Colin Bailey's model has been used for design by a lot of people. He gets some kind of enhancement, membrane enhancement. But what we said is that in a frame like a Cardington situation that this slab is not just sitting on top of a rectangular building without any connection to the rest. It is connected to all the steel beams integrally with these shear studs, so even edge beams. It is continuous, almost it's behaving like it's held by the beams. It cannot just slip out. So we assume that the restraint is coming from those, the boundary restraints, the boundary connections and the continuity in the other parts. So the slab is able to hang. So it's able to hang from those end supports that are where it is tied to. So it's tied in a frame and it's hanging from those and it can hang like that and that membrane action is even more reliable compared to compression ring. Compression ring is generated, that is basically the slab itself doing it. So it kind of is another area that we felt and we use that as a model. We have published some papers. One of my students, neil Cameron, he wrote the first model where we use that analogy of a membrane. But this again the key point to make is on the corner test you were nowhere near the point where the slab was close to failing. It was full of compression, it was just being compressed. The thermal expansion was if something's being pushed against and compressed against supports, it's not going to fall anywhere. It's going to fall when it's really lost its strength and is hanging and then it will start to some unzipping or kind of cracking map appear. But there were no cracking. This did happen in the bigger fourth British filter where you had much larger reflections, almost one meter, okay. And you had much larger reflections that you had cracks. At least at the edges, in the corners you had significant cracks. Okay, these cracks can also be sort of more compatibility related, because it wants to bend and lift up the corners, but the corners are held down, so you get that kind of effect.
Speaker 1:I wonder, because in those tests, in those modeling, you were perhaps for the first time observing this effects with such a detail, giving you such a comprehension of what's happening. And later, later, the life follows and we have some structural failures. Okay, world Tree Center was a very unconventional failure, let's say due to the nature of the fire, but since then we had a lot of classical fires, let's say, like very recent London Luton Car Park fire, which was also, to my best understanding, a steel structure. I'm not sure if you saw that one, but there was also a structural collapse in the frame of that building.
Speaker 2:You heard a bit, but I have not really studied it.
Speaker 1:So I wondered, given this knowledge, have you observed those and confirmed those failure mechanisms, also in large fires, that leading to collapse? Besides World Tree Center, In terms of our.
Speaker 2:One of the things that I've been interested in, which I actually haven't been able to pursue as much as I wanted to, it's the failure of the Gretchenbach parking garage collapse. Do you remember?
Speaker 1:that no In.
Speaker 2:Switzerland.
Speaker 1:Ah, okay, the one that killed some firefighters 2004, killed seven firefighters Okay, Killed seven firefighters.
Speaker 2:And that to me is steel structures. Steel structures. I and then we have been interested in bridge failure. So we've been modeling some of the bridge failures some bridge failure in Oklahoma some of my students have modeled and those are usually like steel bridges and you have this composite behavior, et cetera, and there you do get like simply supported because they're not bridges, they're not link frames, they're actually just sitting, they're just sitting on support so they can actually move.
Speaker 1:You can see the rollers on the bridges.
Speaker 2:Yeah, you can see the rollers, yeah, yeah, so you can get it. You can see them much more easily falling down in a significant fire and there you probably won't get the membrane action that you're talking about, because it just rolls off. So those kind of things we have modeled on the Gretchenbach, which I haven't really been able to study that much. Wanting to, I want to do actually some experiments and I have a suspicion it's never been confirmed. It could be a nice project to do sometime. I have a suspicion. I had a. When I first heard about it, I wrote to the Swiss authorities with a PowerPoint diagram of my hypothesis for the collapse. My hypothesis for the collapse is that what happens in a flat slab situation in a concrete. So it's not steel now, it's concrete. I hope it's still relevant to your situation. Yeah, so if you have a concrete flat sub situation and you have a column impinging on the slab, obviously, and the slab is kind of relatively thicker, it's a flat slab. So you still have this thermal gradient effect. The concrete lower surface of the concrete is much, much hotter temperature than the top surface. There's this huge thermal gradient right and this thermal gradient against the column where it, if you look at the continuity of the column, structural mechanics tells us that that's a point where the rotation is going to be zero because it's like a fixed thing. So at this point the bottom line, just like remember the cardington first test the yield of the steel beam happens at 150 degrees. Low temperature. Yield of the steel beam that means very, very high compression because of this thermal buoying and restraint effect at the bottom, at the lower end of the structure. All the compression is focused at the lower end of the structure. So what? My hypothesis was that this in flat slabs you have this very, very high compression at the lower end or lower surface of the slab and it crushes the concrete. What happens when you crush the concrete near the column, even in the lower layers, if you crush the concrete near that, your whole shear depth is disappearing because your whole slab is just sticking because of that shear force of the shear resistance of the slab. So you take away, you gouge off a significant portion of the bottom through this crushing of the concrete thermal gradient effect. Then you lost significant shear depth of the resistance of the slab and it can punch through. And that's what happened in the Gretchenbach. You had these punched through columns that you can see in the pictures. So okay, they say the load was very high, there was a very significant overload on the. That's what the Swiss first of all the Swiss never replied to me for a long time. They just ignored me. But later on, I think, I found a report which was in Swiss German so I couldn't really read it. So I had to ask some German guy and he said oh, I can't read it, it's Swiss German. So I had to find somebody to sort of give me some what it says. So they said there's, the load was a high. So I think they did some investigation and they blame the loading. I think they had. Just like us, we didn't have any clue when we started doing partying about these thermal effects. So I think they just ignored the thermal effects. They didn't really give it much attention. So I'm not I'm not really just like the many of the World Trade Center investigations are to me, are kind of fantasy, really not really serious. They do a proper job and I think these guys didn't do a proper job either.
Speaker 1:And to close up the story we were having, if you were given today opportunity to approach modeling Cardington, would you still jump in trying to model the whole structure?
Speaker 2:Yeah, well, now I think I've learned from that experience quite a lot and every time I have students who start to take up stuff, I start them with beams. I don't that says stay away from the frames for now, just learn what a beam does. So yeah, I think we don't jump into big frames immediately now, but I think we have more experience now we can do, but once we, once we, but we but students learn quickly then from. We have now this OpenSea software that we use, our own software, rather than Abacus, which is basically this open source software from Berkeley for earthquake engineering, which earthquake engineering people know much more, but not so much the fire community, where we we started adding our the fire simulation, fire thermo mechanical modeling capability in OpenSea in the late 2000s or 2010 or something. We started that work and so now we have many versions of that which we should do quite a good job on very efficiently modeling large frames. We did World Trade Center seven modeling. One of my students finished recently the whole structure modeling the World Trade Center seven full frames, slabs and everything with full frame, with slabs and everything, not the entire building, half of the building or something, and with some stories missing, but still enough to capture the at least the roughly the kind of collapse that have happened here. So in his thesis. But that is too complex so we can't say that that we have really done a proper job on that.
Speaker 1:Okay, okay, now I'm going into selfish mode. I hope my listeners are okay with that. And how do you couple the fire to that? You take CFD like FDS and couple to open.
Speaker 2:So now, yeah, yeah, that's right now. So this is. This is something that we have done quite a lot of work in the last five, six years with two PhD students actually three, okay. So one of them is Dr Artip Ali Khan, who works for University of Canterbury. Now he started I proposed this to when I was in UK. We called it open fire project, where we wanted to connect FDS, the open seas, to sort of automatic fire to heat transfer to, because open heat also has heat transfer. So if you just get the fire, the fluid phase, the fluid phase if you've got a gas temperature of fluxes or whatever into the into the open seas, the heat transfer code, that the heat transfer code will then give you the temperatures and that will just transfer automatic to the structural part, thermo mechanical part, and we can do the thermo. So we have this integrated simulation called an integrated simulation approach. So Artip developed it then and were developed another one. He connected a BIM models with it. So you can have BIM, you can create a BIM model and from that you can you can export files to FDS, export files to open seas and connect them together and get the temperatures automatically from FDS into open seas and do the thermo mechanical analysis. He transferred in thermo mechanical analysis in one in one go. So Anwar is the second student. He went to UQ. He is a lecturer at UQ, artip's lecturer at University of Canterbury, new Zealand. My third student, nan, who's also worked in this area, is going to join Delft in December, the University of Delft as a as also a permanent member of staff, so starting as a postdoc, but I think in three years she will become a, become a tenured assistant professor. So they're doing very well, yeah, so. So if you're interested, the thing is now I'm kind of like supervisor of these guys, so I don't really know how, how they exactly do all of these things.
Speaker 1:Yeah, the tricks are with them.
Speaker 2:So if you, yeah, the tricks are with them. Yeah, yeah, but most of this is all public domain and I also know that open seas is open.
Speaker 1:That's the. It's in the name. The name is open, yeah, but even even what?
Speaker 2:we have done is all open as well. The integrated simulation approach, all the all this work that's been done is all available for anyone who wants to use it for commercial or academic or research purposes.
Speaker 1:Given the background of this software, it could be very interesting to study post-articulate fires with it, if it already has, yeah.
Speaker 2:We have done. We have done also that some students, with some students in UK I did. They do post-earthquake fires. They looked at the dynamic simulation and the loss of the fire protection and then had a fire and the effect of the loss of the fire protection was then. So it was like post-earthquake fire through loss, including the loss of fire protection after earthquake.
Speaker 1:Fantastic. Well, the world of structural fire is really big and interesting, and I love it.
Speaker 2:It tastes quite interesting yeah.
Speaker 1:But we should close on this. So thank you for sharing the story on how. My pleasure how you've modeled the Cardington tests and Thank you for asking me. You're very welcome and I have a feeling we'll see each other a little more in this show.
Speaker 2:Yeah, hopefully, hopefully, yes, yes, you have one of your students is here at these days, so I hope he's behaving good. And he's doing very well.
Speaker 1:Yes, thank you very much, thank you, and that's it. Wow, just wow. This is so interesting to me, like seriously. I'm not an expert on structural fire engineering, my knowledge on the behavior of structures in fires is to some extent basic, and learning all these interesting facts about how thermomechanical response of the structure to thermal load can create conditions in which even materials that are not weakened by the fire can lead to destruction, this is just fascinating to learn and with every episode like this I have more and more respect to the structural engineers. Structural fire engineers, you are doing a fantastic and very difficult and very responsible job and congratulations on doing that. I hope this episode was very interesting to structural engineers. I hope it was very interesting to the general audience as much, because I really wanted to bring this science not just to the top notch structural engineers, who I guess already know as if groundbreaking papers that came from Coddington, but to all of us that are interested in how the hell people make it sure that the structures do not collapse in the case of fire, because every now and then they do. And, like as if mentioned the Wiltryd Center failure or the Gretchenbach car park failure, we still have some catastrophic fires. I find it just great that people can come up with sound models and be able to explain those failures in a way that actually makes sense. So thank you for that. Difficult for me to summarize this anymore because I'm not an expert. I don't want to bring some misconceptions to you, so I'll just say there are papers linked in the show notes and there's also linked to the Tom Lennon's episode, if you'd like to hear about the Coddington experiments on their own Boy. That was a big project, a very interesting ground ranking, unique, one that if you've missed that episode, that's the one that you should listen next. Well, technically you should listen before this episode to have better comprehension, but if you didn't make it, or put it on your playlist and make it the next thing, you listen to the fire science show and regardless of that, I am happy to see you here once again next Wednesday. Thank you Bye. This was the fire science show. Thank you for listening and see you soon.